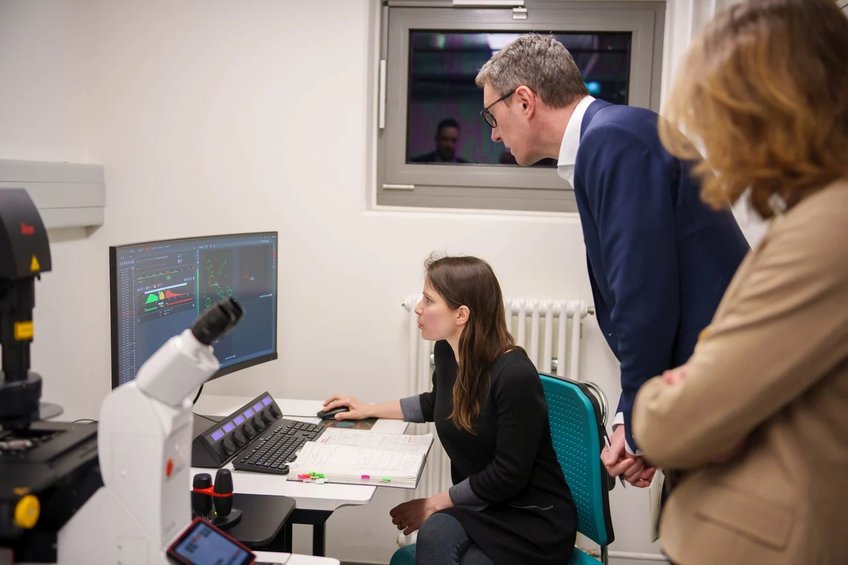
ALLS: Artificial Life-Like Systems
Design of Artificial Cells from Functional Modules
Synthetic Biology is an exciting and rapidly evolving field focused on engineering cells and biological systems to perform predictable and tailored functions—whether modified, enhanced, or entirely novel. The approach involves breaking down the inherent complexity of biological systems into smaller subsystems and functional modules, which are then redesigned and reassembled into larger, integrated units. This methodology is often compared to the design of biotechnological or chemical plants (Rollié et al., 2012). In this context, the PSE Group contributed its expertise in assembling and controlling chemical, energy, and production processes to the MaxSynBio initiative. This research consortium brought together eight Max Planck Institutes, along with research groups from Friedrich-Alexander University Erlangen-Nuremberg and the University of Bordeaux. It was jointly funded by the Max Planck Society and the Federal Ministry of Education and Research in Germany. Building on the achievements of MaxSynBio, the PSE Group’s work in synthetic cell research has progressed into the Artificial Life-like Systems (ALLS) Team, continuing the pursuit of engineered life-like systems with controllable and modular functionalities.
Synthetic Cell Engineering in the ALLS Team
The primary scientific goal of the ALLS Team is to develop a true bottom-up engineering approach (Fig. 1) to mimic the fundamental structural and functional principles of living cells. This approach not only advances our understanding of biological systems but also enables the development of novel synthetic platforms. While the near future of synthetic biology is expected to continue relying largely on top-down strategies (i.e., constructing minimal systems from existing organisms), bottom-up approaches—starting from first principles—offer the potential to refine and enhance these systems using synthetic or alternative biological components, paving the way for a new generation of biotechnological processes.

Researchers in the PSE group have defined the minimal functional modules necessary for creating artificial life-like systems, with a strong focus on inter-module compatibility (Fig. 2). These core functionalities include:
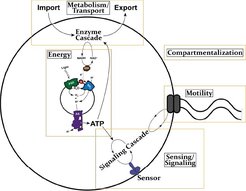
- Motility
- Sensing/Signaling
- Metabolism
- Transport
- Energy generation
For instance, motility enables artificial cells to move in response to environmental stimuli, mimicking key aspects of living organisms. This is tightly integrated with the sensing/signaling module. The energy required for activating motility is supplied in the form of ATP, produced by a metabolic cascade and an ATP-generating module. Enabling technologies such as compartmentalization, membrane transport, cell-free protein expression, and membrane fusion are under continuous development to support these modules.
Importantly, our research is not limited to mimicking natural functions. By integrating natural and synthetic materials, we are uncovering novel and unexpected behaviors in hybrid systems. One key advancement has been the replacement of natural membrane components (e.g., lipids) with synthetic amphiphilic copolymers, which offer broad chemical versatility and tunability for applications in technology, biology, and medicine.
To form vesicles from these synthetic amphiphiles, we are developing and optimizing several fabrication techniques and functionalization protocols, including:
- Gentle film rehydration and extrusion
- Electroformation (and fusion-assisted electroformation)
- Droplet phase transfer
- Microfluidics
For membrane protein functionalization of lipid, polymer, or hybrid membranes, we commonly use:
- Detergent-mediated reconstitution
- Droplet phase transfer
- Membrane fusion
Asymmetric Membranes and Natural-Synthetic Compatibility
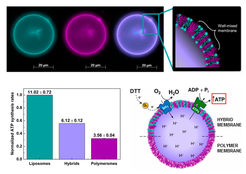

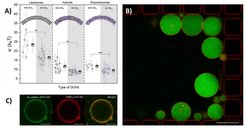
Biological membranes are highly asymmetric, a property essential for functions such as signal transduction. While achieving and maintaining lipid asymmetry is challenging, we explore asymmetric polymer membranes for specific applications. Giant unilamellar vesicles (GUVs) with asymmetric membranes are assembled using either a modified droplet phase transfer method or double-emulsion microfluidics, where each leaflet is formed from a different polymer.
We are particularly interested in the integration of natural components from diverse organisms with synthetic membranes—specifically, the functional behavior of membrane proteins in polymer-based environments (Fig. 3; Otrin et al., 2017; Marušič et al., 2020). We are also exploring various polymer membrane architectures to assess their biocompatibility with natural elements (Fig. 4).
To this end, we have developed tools to study membrane protein activity at the single-vesicle level (Fig. 5), assessing key properties such as: membrane order/disorder, lateral diffusion, thermal stability, and permeability.
Membrane Dynamics and Energy Modules
Understanding membrane dynamics is essential for replicating cellular functions like endocytosis, exocytosis, and division. Our lab explores fusion and fission mechanisms using various strategies, including:
- Mechanical stress
- Salt-induced fusion
- Electrostatic interactions (via charged lipids/polymers)
- Fusogenic peptides (e.g., SNARE proteins)

Active processes in living systems require continuous energy and material supply, tightly linked to cellular metabolism. Following the bottom-up approach, we have assembled and characterized fundamental components for energy conversion and transduction, such as light- and chemically-driven proton pumps and ATP synthase (Fig. 6). We are continuously expanding our library of purified membrane proteins, including oxidases, porins, and fusogenic peptides.
Our expertise includes the assembly and characterization of proteins involved in oxidative phosphorylation and photophosphorylation, including assessments of protein orientation and enzymatic activity. We have also demonstrated cofactor regeneration via encapsulated hydrophobic redox mediators, mimicking the functions of NADH dehydrogenase and cytochrome c reductase (Fig. 7; Wang et al., 2018).

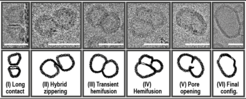
Optimizing membrane protein insertion requires extensive screening of reconstitution conditions, which often vary significantly across different enzymes. To address this, we explore simultaneous integration of multiple enzymes via membrane fusion, utilizing SNARE proteins. Fusion intermediates between polymer and hybrid membranes have also been characterized (Fig. 8; Otrin et al., 2021).
In parallel, we explore metabolic pathways and their integration with energy modules. We also utilize the E. coli respiratory chain as a natural reference system, combining it with minimal synthetic metabolism (Beneyton et al., 2018). Our energy and metabolic modules are further described using mathematical modeling to enable system optimization and provide a quantitative framework for integration.
Finally, to enable targeted localization of signaling and motility modules, we investigate lateral phase separation (Fig. 9; Otrin et al., 2024) in membranes composed of multiple components (e.g., lipids and polymers or polymer blends). We achieve control over microscopically heterogeneous or homogeneous membranes through:
- Membrane composition ratios
- Differences in hydrophobic thickness
- Temperature modulation
- Electrostatic interactions
- Membrane protein integration
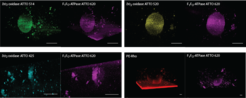
Recent Publications
Otrin N., Otrin L., Bednarz C., Träger T. K., Hamdi F., Kastritis P. L., Ivanov I., and Sundmacher K. (2024). Protein-rich rafts in hybrid polymer/lipid giant unilamellar vesicles. Biomacromolecules 25(2): 778-791
Marušič N., Otrin L., Rauchhaus J., Zhao Z., Kyrilis F.L., Hamdi F., Kastritis P.L., Dimova R., Ivanov I., and Sundmacher K. (2022). Increased efficiency of charge-mediated fusion in polymer/lipid hybrid membranes. Proceedings of National Academy of Sciences 119(20): e2122468119
Marušič N., Zhao Z., Otrin L., Dimova R., Ivanov I., and Sundmacher K. (2022). Fusion-induced Growth of Biomimetic Polymersomes: Behavior of poly(dimethylsiloxane)-poly(ethylene oxide) Vesicles in Saline Solutions Under High Agitation. Macromolecular Rapid Communications 43(5): e2100712
Staufer O., De Lora J. A., Bailoni E., Bazrasfhan A., Benk A. S., Jahnke K., Manzer Z. A., Otrin L., Pérez T. D., Sharon J., Steinkühler J., Adamala K. P., Jacobson B., Dogterom M., Göpfrich K., Stefanovic D., Atlas S. R., Grunze M., Lakin M. R., Shreve A. P., Spatz J. S., and López G. P. (2021). Science Forum: Building a community to engineer synthetic cells and organelles from the bottom-up. eLife 10: e73556
Otrin L., Witkowska A., Marušič N., Zhao Z., B. Lira R., Kyrilis F., Hamdi F., Ivanov I., Lipowsky R., L. Kastritis P., Dimova R., Sundmacher K., Jahn R., and Vidaković-Koch T. (2021). En route to dynamic life processes by SNARE-mediated fusion of polymer and hybrid membranes. Nature Communications 12: 4972
Shetty C. S., Yandrapalli N., Pinkwart K., Krafft D., Vidakovic-Koch T., Ivanov I., and Robinson T. (2021). Directed Signaling Cascades in Monodisperse Artificial Eukaryotic Cells. ACS Nano 15: 15656−15666
Balasbas III S., Ivanov I. and Sundmacher K. (2021). Metabolic Network Reconstruction from Time-Series of Concentration Data: Evaluation of the Numerical Matrices Methods. Computer Aided Chemical Engineering 50: 1991-1996
Ivanov I., López Castellanos S., Balasbas III S., Otrin L., Marušič N., Vidaković-Koch T., and Sundmacher K. (2021). Bottom-Up Synthesis of Artificial Cells: Recent Highlights and Future Challenges. Annual Review of Chemical and Biomolecular Engineering 12: 287-308
Wang M., Weber A., Hartig R., Zheng Y., Krafft D., Vidaković-Koch T., Zuschratter W., Ivanov I., Sundmacher K. (2021). Scale up of transmembrane NADH oxidation in synthetic giant vesicles. Bioconjugate Chemistry 32 (5): 897–903.
Ahmad R., Kleineberg C., Nasirimarekani V., Su Y.-J., Pozveh S. G., Bae A., Sundmacher K., Bodenschatz E., Guido I., Vidaković-Koch T., and Gholami A. (2021). Light-Powered Reactivation of Flagella and Contraction of Microtubule Networks: Toward Building an Artificial Cell. ACS Synthetic Biology 10 (6): 1490–1504
Wohlfromm F., Richter M., Otrin L., Seyrek K., Vidaković-Koch T., Kuligina E., Richter V., Koval O., and Lavrik I. N. (2021). Interplay Between Mitophagy and Apoptosis Defines a Cell Fate Upon Co-treatment of Breast Cancer Cells With a Recombinant Fragment of Human κ-Casein and Tumor Necrosis Factor-Related Apoptosis-Inducing Ligand. Frontiers in Cell and Developmental Biology 8: 617762
Xu D., Kleineberg C., Vidaković-Koch T., Wegner S. V. (2020). Multistimuli sensing adhesion unit for the self-positioning of minimal synthetic cells. Small 16 (35): 2002440
Marušič N., Otrin O., Zhao Z., Lira R.B., Kyrilis F.L., Hamdi F., Kastritis P.L., Vidaković-Koch T., Ivanov I., Sundmacher K., and Dimova R. (2020). Constructing artificial respiratory chain in polymer compartments: Insights into the interplay between bo3 oxidase and the membrane. Proceedings of National Academy of Sciences 117 (26): 15006-15017
Kleineberg C., Wölfer C., Abbasnia A., Pischel D., Bednarz C., Ivanov I., Heitkamp T., Börsch M., Sundmacher K., Vidaković-Koch T. (2020). Light-driven ATP regeneration in diblock/grafted hybrid vesicles. ChemBioChem 21 (15): 2149-2160
Otrin L., Kleineberg C., de Silva L.C., Landfester K., Ivanov I., Wang M, Bednarz C., Sundmacher K., Vidaković-Koch T. (2019). Artifical organelles for energy regeneration. Advanced Biosystems 3 (6): 1800323
Ivanov I., Lira R.B., Tang T.-Y.D., Franzmann T., Klosin A., de Silva L.C., Hyman A., Landfester K., Lipowsky R., Sundmacher K., and Dimova R. (2019). Directed growth of biomimetic microcompartments. Advanced Biosystems 3: 1800314
Krafft D., López Castellanos S., Lira R.B., Dimova R., Ivanov I., Sundmacher K. (2019). Compartments for synthetic cells: Osmotically assisted separtion of oil from double emulsions in microfluidic chip. ChemBioChem 20: 2604-2608
Beneyton T., Krafft D., Bednarz C., Kleineberg C.,Woelfer C., Ivanov I., Vidaković-Koch T., Sundmacher K., and Baret J. C. (2018). Out-of-equilibrium microcompartments for the bottom-up integration of metabolic functions. Nature Communications 9: 2391
Wang M., Wölfer C., Otrin L., Ivanov I., Vidaković-Koch T., and Sundmacher K. (2018). Transmembrane NADH Oxidation with Tetracyanoquinodimethane. Langmuir 34 (19): 5435-5443
Weiss M., Frohnmayer J. P., Benk L. T., Haller B., Janiesch J. W., Heitkamp T., Börsch M., Lira R. B., Dimova R., Lipowsky R., Bodenschatz E., Baret J. C., Vidakovic-Koch T., Sundmacher K., Platzman I., Spatz J. P. (2018). Sequential bottom-up assembly of mechanically stabilized synthetic cells by microfluidics. Nature Materials 17(1): 89-96
Schwille P., Spatz J., Landfester K., Bodenschatz E., Herminghaus S., Sourjik V., Erb T. J., Bastiaens P., Lipowsky R., Hyman A., Dabrock P., Baret J. C., Vidaković-Koch T., Bieling P., Dimova R., Mutschler H., Robinson T., Tang T. D., Wegner S., and Sundmacher K. (2018). MaxSynBio: Avenues Towards Creating Cells from the Bottom Up. Angewandte Chemie International Edition 57 (41):13382-13392
Otrin L., Marušič N., Bednarz C., Vidaković-Koch T., Lieberwirth I., Landfester K., and Sundmacher K. (2017). Toward Artificial Mitochondrion: Mimicking Oxidative Phosphorylation in Polymer and Hybrid Membranes. Nano Letters 17 (11): 6816-6821
Rollié S., Mangold M. and Sundmacher K. (2012). Designing biological systems: systems engineering meets synthetic biology. Chemical Engineering Science, 69 (1): 1-29
Collaborations
Natural Building Blocks
Prof. Volker Zickermann, Medical Faculty of Goethe University, Frankfurt, Germany
Prof. Marc Nowaczyk, Ruhr University Bochum, Bochum, Germany
Prof. Sonja-Verena Albers, University of Freiburg, Freiburg, Germany
Synthetic Building Blocks
Prof. Sebastién Lecommandoux, Université de Bordeaux, Bordeaux, France
Prof. César Rodriguez-Emmenegger, Institute for Bioengineering of Catalonia, Barcelona, Spain
Biophysical and Structural Analysis of Vesicles
Prof. Panagiotis L. Kastritis, Martin Luther University Halle-Wittenberg, Halle, Germany
Prof. Katharina Landfester, Max Planck Institute for Polymer Research, Mainz, Germany
Dr. Rumiana Dimova, Prof. Reinhard Lipowsky, Max Planck Institute of Colloids and Interfaces, Potsdam, Germany