Multidimensional Crystallization Processes
Worldwide about 60% of the chemical production is connected with solid materials. Very often, crystallization from solution is one important step during the process, for separation, for purification, for maintaining specific product properties. The industrial production of large amounts of product is mostly done in continuous processes, therefore a continuous crystallization is applied, but it requires a thorough understanding of the physical and chemical phenomena involved.
In our research on continuous crystallization we have developed two model crystallizers: (1) a flow-through tube reactor where crystallization of solid particles takes places inside a fluid moving along a tube with a variable residence time inside a particularly defined flow field and (2) a fluidized-bed crystallizer with variable inlet and outlet conditions and a controllable flow-field pattern (see Fig. 1).
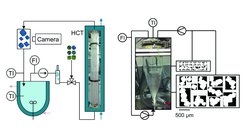
We investigated in detail applying our own image processing and analysis methods [1,2,3] how the crystal shape changes during different types of crystallization. Understanding these details lead us to methods to control the specific shapes or to approaches how to induce specific and desired crystal shape changes [4,5,6]. Part of this work was done in collaboration with partners from WIAS Berlin (Prof. John) and from TUH Hamburg (Prof. LeBorne), under the umbrella of the SPP 1679 “Dynamic simulation of interconnected solid particle processes”. Flow-field calculations by Prof. John and efficient mathematical methods to solve complex population balance equations by Prof. LeBorne were combined with our experimental model crystallization imaging and process data to obtain new insights into these complex processes [7,8].
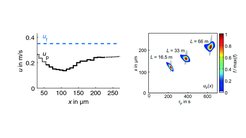
One important outcome of our flow-tube experiments is the measured crystal-size dependent travel velocity and the corresponding reciprocal residence time of crystals in setup (1). Combined with model simulation we showed that such behavior leads to size-related narrowing of crystal populations (see Fig. 2), a result which is very useful in applications as narrow-sized products are often the desired outcome.
Based on our experience in crystallization and precipitation we will focus in the next years on a new collaboration with Prof. Wilcox from the University of Pennsylvania, USA where we study the optimal route towards one viable carbon capture and storage (CCS) option, namely using waste mine tailings and CO2 to precipitate solid carbonates for long-time storage of this climate-changing greenhouse gas [9]. The collaboration has already started and first experiments have shown the great potential of CO2 mineralization for carbon capture and storage (see Fig.3).
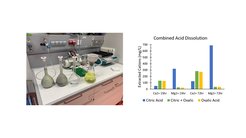
Publications
[1] Borchert, C. and Sundmacher, K. (2011). Crystal aggregation in a flow tube: Image-based observation. Chemical Engineering Technology, 34, 545-556.
[2] Eisenschmidt, H., Voigt, A. and Sundmacher, K. (2015). Face-specific growth and dissolution kinetics of potassium dihydrogen phosphate crystals from batch crystallization experiments. Crystal Growth & Design, 15, 219-227.
[3] Temmel, E., Eisenschmidt, H., Lorenz, H., Sundmacher, K. and Seidel-Morgenstern, A. (2016). A short-cut method for the quantification of crystallization kinetics. 1. Method development, Crystal Growth & Design, 16, 6743-6755.
[4] Wiedmeyer, V., Voigt, A. and Sundmacher, K. (2017). Crystal population growth in a continuous helically coiled flow tube crystallizer. Chemical Engineering & Technology, 40, 1584-1590.
[5] Wiedmeyer, V., Anker, F., Bartsch, C., Voigt, A., John, V. and Sundmacher, K. (2017). Continuous Crystallization in a Helically Coiled Flow Tube: Analysis of Flow Field, Residence Time Behavior, and Crystal Growth. Industrial & Engineering Chemistry Research, 56 (13), 3699-37
[6] Wiedmeyer, V. Voigt, A., Anker, F., John, V. Ahrens, R., LeBorne, S. and Sundmacher, K. (2018). Kontinuierliche formselektive Kristallisation: Neue Strategien, Chemie Ingenieur Technik 9, p. 1198.
[7] Bartsch, C., Wiedmeyer, V. Lakdawala, Z., Patterson, R.I.A., Voigt, A. Sundmacher K. and John, V. (2019). Stochastic-Deterministic Population Balance Modeling and Simulation of a Fluidized Bed Crystallizer Experiment, Chemical Engineering Science, 208, 115102.
[8] Ahrens, R., Lakdawala, Z., Voigt, A., Wiedmeyer, V., John, V., LeBorne, S. and Sundmacher, K. (2020). Numerical Methods for Coupled Population Balance Systems Applied to the Dynamical Simulation of Crystallization Processes, in: Dynamic Flowsheet Simulation of Solids Processes (Editor: S. Heinrich), Book Chapter 14, p.475-518.
[9] Woodall, C. (2021). A Multi-Faceted Approach to Carbon Mineralization Advancement, PhD Dissertation, Worchester Polytechnic Institute, p. 41-65.