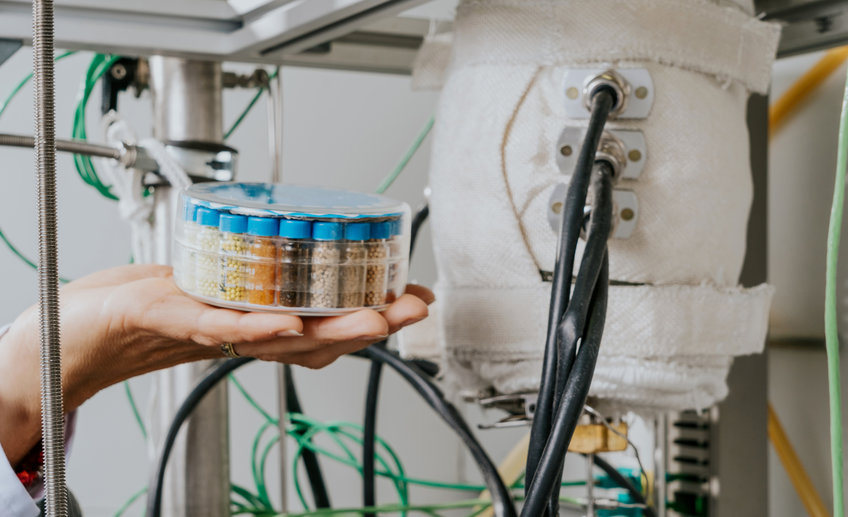
Dynamic operation
The effective control and use of dynamic reactor operation is essential to enhance the desired performance parameters. Dynamic operation can be either systemic, i.e. catalyst deactivation, or forced, modulation of inlet parameters. With the application of suitable methodologies, it is possible to determine dynamic operating parameters that enhance a process compared to their steady state operation. Further, dynamic operation of multifunctional reactors can be used to reduce downtimes compared to conventional reactor concepts.
Nonlinear Frequency Response Method
In chemical processes it is usually desired to prevent dynamic behavior of the system. Even though, it was proven that suitable dynamic parameters result in beneficial performance. The application of theoretical results of forced periodic operation has been limited, because of inaccurate predictability.
A relatively new and fast method to evaluate dynamic reactor operation is the nonlinear frequency response (NFR). This method, based on control theory, predicts the output of forced periodically operating single and multiple input parameters (Figure 1), such as inlet concentration, total flow and inlet temperature. In a collaboration with the University of Belgrade sinusoidal changes and more general waveforms of these inputs are being validated.
![Figure 1: Schematic illustration inputs modulating resulting in a fluctuating output, which is beneficial when the time-average is higher compared to the steady state case for the product.[Felischak, M., Kaps, L., Hamel, C., Nikolic, D., Petkovska, M., & Seidel-Morgenstern, A. (2021). Analysis and experimental demonstration of forced periodic operation of an adiabatic stirred tank reactor: Simultaneous modulation of inlet concentration and total flow-rate. Chemical Engineering Journal, 128197.]](/4224743/original-1636969253.jpg?t=eyJ3aWR0aCI6MjQ2LCJvYmpfaWQiOjQyMjQ3NDN9--68a362bf3f458f4861b9e70d36b896ac93b94db5)
[Felischak, M., Kaps, L., Hamel, C., Nikolic, D., Petkovska, M., & Seidel-Morgenstern, A. (2021). Analysis and experimental demonstration of forced periodic operation of an adiabatic stirred tank reactor: Simultaneous modulation of inlet concentration and total flow-rate. Chemical Engineering Journal, 128197.]
The NFR method is based on the concept of higher order frequency response functions (FRFs) and is applicable for weakly nonlinear systems. Frequency response of a weakly nonlinear system, in addition to the basic harmonic, contains a non-periodic (DC) term and, theoretically, an infinite sequence of higher harmonics. The DC component of the output is responsible for the average performance of the periodically operated reactor, and its sign and value define whether, and to which extent, the periodic operation leads to process improvement.
Forced Simultaneous Modulation of Multiple Reactor Inlet Parameter
As an example, the hydrolysis of acetic anhydride in an adiabatic CSTR has been chosen. In order to implement single and multiple input parameter perturbations an experimental setup was built.
It was possible to show that the single input modulation does not change the time-average output, in case of inlet concentration, or results in detrimental output, in case of total flow perturbation. For the simultaneous modulation of inlet concentration and total flow, the phase difference between the two inlets is the essential parameter to obtain beneficial operating conditions, in comparison to the steady-state. This is presented in Figure 2 and the corresponding inlet modulating function in Figure 3. A clear maximum is observed at the optimal phase difference.
![Figure 2: Experimental and simulative representation of simultaneous variation of the inlet concentration and volumetric flow rate for the hydrolysis of acetic anhydride with fixed amplitudes and varying phase shift.[Felischak, M., Kaps, L., Hamel, C., Nikolic, D., Petkovska, M., & Seidel-Morgenstern, A. (2021). Analysis and experimental demonstration of forced periodic operation of an adiabatic stirred tank reactor: Simultaneous modulation of inlet concentration and total flow-rate. Chemical Engineering Journal, 128197.]](/3548671/original-1636969253.jpg?t=eyJ3aWR0aCI6MjQ2LCJvYmpfaWQiOjM1NDg2NzF9--bbfe24945a7460de84d8267d38e8dd4de166e7ef)
[Felischak, M., Kaps, L., Hamel, C., Nikolic, D., Petkovska, M., & Seidel-Morgenstern, A. (2021). Analysis and experimental demonstration of forced periodic operation of an adiabatic stirred tank reactor: Simultaneous modulation of inlet concentration and total flow-rate. Chemical Engineering Journal, 128197.]
Based on these highly promising and worldwide first performed experiments the NFR method is applied to the industrially relevant methanol synthesis. This heterogeneously catalyzed reaction is performed at pressures of 30-60 bar and 230-260 °C adding to the complexity of implementing forced dynamic operation (Figure 3).
![Figure 3: Reaction network for the methanol synthesis for simultaneous feed of carbon monoxide and carbon dioxide.[Seidel, C., Jörke, A., Vollbrecht, B., Seidel-Morgenstern, A., & Kienle, A. (2018): Kinetic modeling of methanol synthesis from renewable resources. Chemical Engineering Science, 175, 130-138.]](/3548769/original-1636969253.jpg?t=eyJ3aWR0aCI6MjQ2LCJvYmpfaWQiOjM1NDg3Njl9--153de051e4993609769fbbfa2007d4df707ab8c4)
[Seidel, C., Jörke, A., Vollbrecht, B., Seidel-Morgenstern, A., & Kienle, A. (2018): Kinetic modeling of methanol synthesis from renewable resources. Chemical Engineering Science, 175, 130-138.]
To realize this challenging process a modular experimental setup in a mini plant scale was assembled with a Berty-type reactor as the centerpiece for realizing ideal CSTR conditions within the reactive zone (Figure 4).
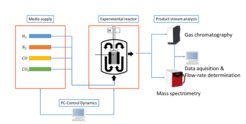
First results of the NFR method present interesting results to the optimized steady-state operation that are in good agreement with a rigorous optimization (Figure 5). Validation of the NFR results with rigorous numerical optimization for a minimal periodic time of 18 s.
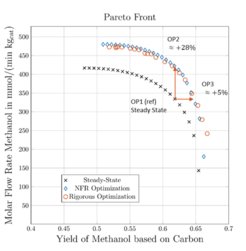
References
Seidel, C., Nikolić, D., Felischak, M., Petkovska, M., Seidel-Morgenstern, A., & Kienle, A. (2021). Optimization of methanol synthesis under forced periodic operation. Processes 9(5), 872.
Felischak, M., Kaps, L., Hamel, C., Nikolic, D., Petkovska, M., & Seidel-Morgenstern, A. (2021). Analysis and experimental demonstration of forced periodic operation of an adiabatic stirred tank reactor: Simultaneous modulation of inlet concentration and total flow-rate. Chemical Engineering Journal, 128197.
Nikolić, D., Seidel, C., Felischak, M., Miličić, T., Kienle, A., Seidel-Morgenstern, A., & Petkovska, M. (2021). Forced periodic operations of a chemical reactor for methanol synthesis – the search for the best scenario based on NFR method. Part I Single input modulations. Chemical Engineering Science, 248, 117134.
Nikolić, D., Seidel, C., Felischak, M., Miličić, T., Kienle, A., Seidel-Morgenstern, A., & Petkovska, M. (2021). Forced periodic operations of a chemical reactor for methanol synthesis – the search for the best scenario based on NFR method. Part II Simultaneous modulation of two inputs. Chemical Engineering Scince, 248, 117133.
Nikolić, D., Seidel-Morgenstern, A., & Petkovska, M. (2020). Nonlinear frequency response analysis of forced periodic operations with simultaneous modulation of two general waveform inputs with applications on adiabatic CSTR with square-wave modulations. Chemical Engineering Science, 226: 115842.
Seidel, C., Jörke, A., Vollbrecht, B., Seidel-Morgenstern, A., & Kienle, A. (2020). Corrigendum to “Kinetic modeling of methanol synthesis from renewable resources” (Chem. Eng. Sci. 175 (2018) 130-138). Chemical Engineering Science, 223: 115724.
Seidel, C., Jörke, A., Vollbrecht, B., Seidel-Morgenstern, A., & Kienle, A. (2018). Kinetic modeling of methanol synthesis from renewable resources. Chemical Engineering Science, 175, 130-138.
Seidel, C., Jörke, A., Vollbrecht, B., Seidel-Morgenstern, A., & Kienle, A. (2018). Kinetic Modeling of Methanol Synthesis - Impact of Catalyst Deactivation. In 28th European Symposium on Computer Aided Process Engineering, 85-90.
Petkovska, M., Nikolić, D., & Seidel-Morgenstern, A. (2018). Nonlinear Frequency Response Method for Evaluating Forced Periodic Operations of Chemical Reactors. Israel Journal of Chemistry, 58(6-7), 663-681.
Membrane Reactors for Integrated Coupling of Oxidative and Thermal Dehydrogenation of Propane
Besides forced dynamic behavior of chemical reactors, a lot of catalytic chemical processes show unwanted dynamic behavior due to catalyst deactivation. One way to enhance the performance of such processes is to use other, more advanced catalysts. Another way is to develop integrated multifunctional reactor concepts and strategies for a dynamic operation, including catalyst regeneration, and to avoid reactor down times.
An industrial relevant reaction that shows rapid catalyst coking is the thermal dehydrogenation of propane (TDH, Figure 1, R5). This well-established reaction for on-purpose production of propene is especially interesting since the product range of crackers shifts to ethene due to the abundance of shale gas as feedstock. The TDH is used in different commercialized processes (CATOFIN®/STAR®/Oleflex® process) and shows a high selectivity towards propene. An alternative reaction is the oxidative dehydrogenation (ODH, Figure 1, R1) which is less efficient due to unwanted side reactions, e.g. total oxidation, but shows no coking and higher propane conversion.
![Figure 1: Reaction network of the ODH and TDH[Walter, J. P., Brune, A., Seidel-Morgenstern, A., & Hamel, C. (2021). Process Intensification of the Propane Dehydrogenation Considering Coke Formation, Catalyst Deactivation and Regeneration—Transient Modelling and Analysis of a Heat-Integrated Membrane Reactor.]](/3549170/original-1636648814.jpg?t=eyJ3aWR0aCI6MjQ2LCJvYmpfaWQiOjM1NDkxNzB9--6bb69bbee06eb1b442bbebd071a82e71d66a26dd)
[Walter, J. P., Brune, A., Seidel-Morgenstern, A., & Hamel, C. (2021). Process Intensification of the Propane Dehydrogenation Considering Coke Formation, Catalyst Deactivation and Regeneration—Transient Modelling and Analysis of a Heat-Integrated Membrane Reactor.]
The combination and thermal integration of oxidative and thermal dehydrogenation in one multifunctional membrane reactor can help to overcome these disadvantages, leading to higher propene yield and a more effective process. This integration is investigated in the project „Kontrolle und Intensivierung von Reaktionen durch Einsatz zyklisch betriebener Distributoren“ (SE 568/23-1 / HA 6762/2-1), supported by the German Research Foundation.
However, the optimization of conversion and selectivity is an ambitious as well as complex challenge and several process designs and degrees of process integration are thinkable. Regardless of the process setup, coking of the catalyst leads to the necessity of a dynamic operation. To overcome reactor down times, the highly integrated membrane reactor concept with internal reflux and thermal integration offers the potential of operando regeneration of one catalyst bed, while the dehydrogenation takes place in an integrated second catalyst bed (Figure 2).
![Figure 2: Coupling of TDH and ODH in a membrane reactor including operando regeneration by flow reversal.[Brune, A., Wolff, T., Seidel-Morgenstern, A., & Hamel, C. (2019). Analysis of Membrane Reactors for Integrated Coupling of Oxidative and Thermal Dehydrogenation of Propane. Chemie Ingenieur Technik, 56(2), 251.]](/3550072/original-1636648814.jpg?t=eyJ3aWR0aCI6MjQ2LCJvYmpfaWQiOjM1NTAwNzJ9--d7b82db0ef570cbe3536a3b9c8a8757271f0d21c)
[Brune, A., Wolff, T., Seidel-Morgenstern, A., & Hamel, C. (2019). Analysis of Membrane Reactors for Integrated Coupling of Oxidative and Thermal Dehydrogenation of Propane. Chemie Ingenieur Technik, 56(2), 251.]
For both TDH and ODH a VOx catalyst (Al2O3 support) has been used. Experiments with different types of integrated reactors have shown a better performance than established fixed bed reactors (Figure 3). Especially the use of CO2 as a reactive sweep gas seems to be promising to shift the chemical equilibrium in the water gas shift reaction (Figure 1, R4), overcoming the thermodynamically limited conversion of the TDH.
![Figure 3: Comparison of different membrane reactor setups (extractor vs. distributor) at different temperatures (WHSV =400 kg s m-3, xC3H8 in = 1%).[Brune, A., Wolff, T., Seidel-Morgenstern, A., & Hamel, C. (2019). Analysis of Membrane Reactors for Integrated Coupling of Oxidative and Thermal Dehydrogenation of Propane. Chemie Ingenieur Technik, 56(2), 251.]](/3550099/original-1636648814.jpg?t=eyJ3aWR0aCI6MjQ2LCJvYmpfaWQiOjM1NTAwOTl9--b1d9326deec87167a7a61a5d0cf18b97c557fa6f)
[Brune, A., Wolff, T., Seidel-Morgenstern, A., & Hamel, C. (2019). Analysis of Membrane Reactors for Integrated Coupling of Oxidative and Thermal Dehydrogenation of Propane. Chemie Ingenieur Technik, 56(2), 251.]
For a better understanding of TDH and ODH in one apparatus the developing concentration and temperature fields have to be studied simultaneously. Due to coupling of heat and mass balances, a simulation of integrated reactors is not trivial. Kinetic parameters for the desired and undesired reactions have to be available. This includes deactivation kinetics and regeneration kinetics, respectively. To investigate the reaction network, experiments in a fixed bed reactor have been conducted. The deactivation and regeneration kinetics have been studied in a TGA. A detailed modeling of all processes allows to simulate and optimize the overall production process of a PFTR in 1D simulations especially with regard to an optimized length of the production and regeneration phases. Based on the derived kinetic parameters 2D-simulations of reactor setups with different degrees of process integration are possible to evaluate the potential of multifunctional reactors (Figure 4).
Consequently, a comprehensive kinetic study of the model reactions allows to identify beneficial process conditions for new reactor concepts. The models and kinetic parameters can serve as a basis for the design and intensification of cyclically operating deactivation and regeneration processes involving catalyst coking. This allows to evaluate more complex reactor geometries, established production processes for the conversion of propane to propene (Catofin, Oleflex, ...), innovative reactor concepts, and advanced regeneration strategies.
![Figure 4: Calculated 2D profiles of propene in a heat-integrated membrane reactor at different times (considering catalyst deactivation); W/F = 400 (kg s)/m³, C3H8in = 1 %, O2/C3H8 = 1, Tin = 600 °C, Comsol.[ Walter, J. P., Brune, A., Seidel-Morgenstern, A., & Hamel, C. (2021). Process Intensification of the Propane Dehydrogenation Considering Coke Formation, Catalyst Deactivation and Regeneration—Transient Modelling and Analysis of a Heat-Integrated Membrane Reactor.]](/3549488/original-1636648814.jpg?t=eyJ3aWR0aCI6MjQ2LCJvYmpfaWQiOjM1NDk0ODh9--7f2fcd59123243ec258b3dfbaedbfe7232d620be)
[ Walter, J. P., Brune, A., Seidel-Morgenstern, A., & Hamel, C. (2021). Process Intensification of the Propane Dehydrogenation Considering Coke Formation, Catalyst Deactivation and Regeneration—Transient Modelling and Analysis of a Heat-Integrated Membrane Reactor.]
References
Brune, A., Geschke, A., Seidel-Morgenstern, A., & Hamel, C. (2021). Modeling and Simulation of Catalyst Deactivation and Regeneration Cycles for Propane Dehydrogenation - Comparison of Different Modeling Approaches. Chemical Engineering and Processing: Process Intensification, 108689.
Walter, J. P., Brune, A., Seidel‐Morgenstern, A., & Hamel, C. (2021). Model‐based Analysis of Fixed‐bed and Membrane Reactors of Various Scale. Chemie Ingenieur Technik, 93(5), 819–824.
Brune, A., Seidel-Morgenstern, A., & Hamel, C. (2020). Analysis and Model-Based Description of the Total Process of Periodic Deactivation and Regeneration of a VOx Catalyst for Selective Dehydrogenation of Propane. Catalysts, 10(12), 1374.