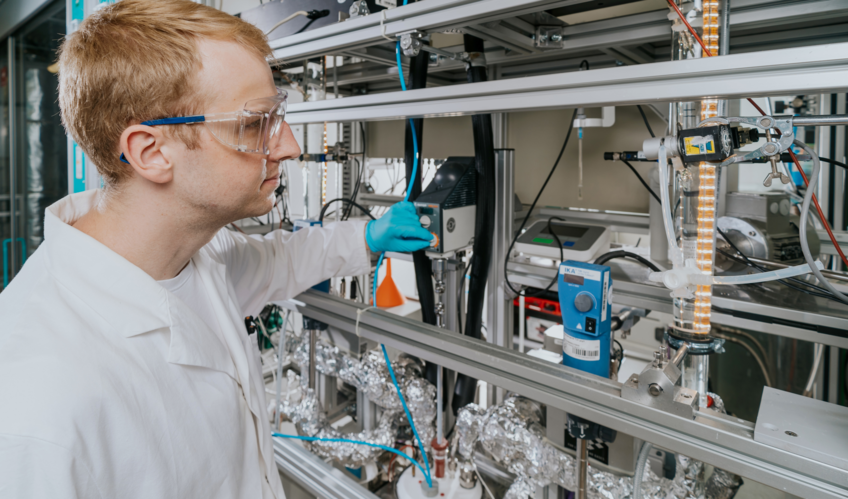
Process design
An important aspect of the crystallization-related research of our group is the development of advanced and alternative process concepts for separation problems. This concerns separation tasks where one specific target component has to be isolated from a complex multi-component mixture (such as a drug molecule out of a plant extract), and also cases where two components have to be separated which are hardly resolvable by crystallization (such as enantiomers and other very similar isomers). The development of particularly sustainable crystallization processes for carbon-neutral production concepts is also of special importance for us.
Process variants studied refer to continuous, multistage and batch operation modes, advanced process strategies for enantiomer separation, separation concepts for solid solutions and the recovery of solid lignin (-fractions) from organosolv pulping liquors.
Feasibility, yield, productivity and product properties achievable with the different process options are examined in the related projects. In addition, the potential applicability of the concepts at industrial scale is evaluated. The application of advanced process analytical techniques and rationalization of process design are also considered.
Crystallization for carbon-neutral production processes: CODA project
Many bulk products in chemical industry are crystalline and are produced in crystallization processes, which can have a significant impact on the energy consumption and thus the carbon-footprint of the product. Our goal is therefore to develop carbon-neutral crystallization processes with minimum energy requirements that can be driven by renewable energies. Additionally, we aim for closed material and solvent cycles in order to reduce the environmental impact and improve economy of these sustainable processes.
In the CODA (Carbon-negative sODA ash plant) project (https://www.fona.de/en/measures/funding-measures/KlimPro/coda.php), a new sustainable and carbon-negative process for the production of calcined soda ash (sodium carbonate) is being developed.
The project is directed to the development of a new process for the production of soda ash using atmospheric CO2 by direct air capture (DAC) and caustic soda generated by electrolysis of rock salt brine using renewable electrical energy. Hydrogen and chlorine are produced as valuable by-products of the electrolysis (Figure 1). A particular challenge in the development of the CODA process is the strong dependence of the process on the weather conditions. The generation of renewable electricity from the sun and wind is just as dependent on the weather conditions as the CO2 DAC from atmospheric humidity and temperature.
Therefore, especially the crystallization process must be adaptable to a range of feed compositions from the DAC process. Variations in water content and solids load have to be considered in the process development. Heat integration is of great importance to minimize energy demand. Mother liquor and solvent will be recycled to the greatest extend to minimize waste streams.
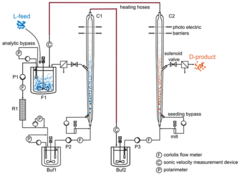
Crystallization concepts for separation of enantiomers
Each enantiomer of a pair presents different pharmacological activity and there is an increasing demand for producing pure chiral molecules. Enantiomer separation can be achieved by two main approaches: asymmetric synthesis or nonselective synthesis followed by chiral resolution. A great effort has been done to develop separation techniques to obtain pure enantiomers. Crystallization is an attractive process since it directly provides a solid product, as frequently desired form for pharmaceuticals.
Preferential Crystallization of conglomerate forming systems is a selective method that can applied as separation technique in the area of the pharmaceutical, agricultural and food industries. The classical single batch approach, in which one crystallizer is seeded with homochiral crystals is an inherently low-robust process and suffers from strong yield limitations. The challenge is to overcome this limitations not only by using a continuous approach but also keeping the process in batch configuration.
One of the configurations that can be used in order to increase the performance of the process consists in Coupling Preferential Crystallization with selective Dissolution (CPCD) using two coupled stirred tanks operating at different temperatures (Figure 2). The application of online/inline measurements techniques to monitor the progress of the resolution with time allows to control the process parameters in both tanks. This method is capable of providing robustly both pure enantiomers from racemic mixtures introducing only a small amount of seeds of one of the enantiomers.
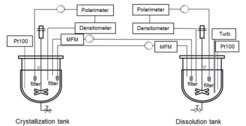
The population balance model (PBM) (Equation 1) describes particulate processes and it has been frequently applied to model and optimize Preferential Crystallization.

It incorporates the various mechanisms involved in crystallization, e.g. growth, nucleation, breakage, agglomeration. Due to the consideration of many details, the resulting set of equations is relatively difficult to solve and expensive in applying it for simulation and optimization. Many studies have been made to ease experimental and computational efforts, and to enable systematic implementation and efficient usage of the PBM. A shortcut model (SCM) to describe Preferential Crystallization based on lumped crystallization mechanisms is developed to facilitate more rapid analysis of important process design parameters. The model is capable to describe main features of isothermal batch Preferential Crystallization using only three differential equations, which are formulated from the mass balances of the preferred enantiomer in the liquid and solid phases. To expand the applicability of the model to continuous Preferential Crystallization, a well established knowledge of chemical reaction engineering has been used. The well-known analogy between batch processes performed in perfectly mixed tanks and continuous processes performed in tubes under plug flow conditions is applied to develop generally valid dimensionless equations for the SCM given in equations 2-4.
Liquid phase balance:

Solid phase balance:
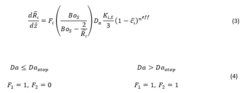
Continuous strategies can often improve the process performance in terms of productivity, product quality as well as in terms of reproducibility. The choice of an appropriate concept of continuous crystallization depends, for example, on the product specific aims and requirements.
Current work is directed to the application of Coupled Fluidized Bed Crystallizers (CFBC) for the continuous separation of enantiomers. Main focus is here a high product purity, why it is attractive for the production of active pharmaceutical ingredients (APIs). The example systems studied originate from the life-science and pharmaceutical industries.
The CFBC combines the principle of fluidized bed crystallization with the technique of preferential crystallization (Figure 3). Therefore the CFBC uses two conical shaped tubular crystallizers, which both are seeded with one of the pure enantiomers. The solid racemic mixture to be separated is dispersed in a corresponding saturated solution within a shared feed tank. The crystal-free saturated solution is pumped continuously from the bottom through the tubular crystallizers, while cooling is provided via double-wall jackets. To ensure continuous selective seeding, grown enantiopure crystals are withdrawn at the bottom of the crystallizers, fragmented in a bypass and fed back as seeds to the crystallizers.
The conical shape of the tubular crystallizer causes a varying fluid velocity over the crystallizer height, which results in a classifying effect on the crystals. Hence, the application of CFBC shows particular beneficial features.
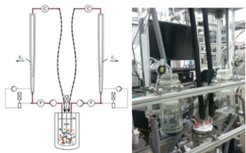
References
Gänsch, J., Huskova, N., Kerst, K., Temmel, E., Lorenz, H., Mangold, M., Janiga, & G., Seidel-Morgenstern, A. (2021). Continuous Enantioselective Crystallization of Chiral Compounds in Coupled Fluidized Beds, Chemical Engineering Journal. 422, 129627.
Huskova, N., Mangold, M., Seidel-Morgenstern, A. (2021). Optimization of a Continuous Fluidized Bed Process for the Separation of Enantiomers by Preferential Crystallization, Computer Aided Process Engineering, 50, 381-386.
Mangold, M., Huskova, N., Gänsch, J., & Seidel-Morgenstern, A. (2020). Linear Analysis of a Continuous Crystallization Process for Enantiomer Separation. Processes, 8, 1337.
Cascella, F., Temmel, E., Seidel-Morgenstern, A., & Lorenz, H. (2020). Efficient resolution of racemic guaifenesin via batch preferential crystallization processes. Organic Process Research & Development, 24(1), 50-58.
Temmel, E., Gänsch, J., Seidel-Morgenstern, A., & Lorenz, H. (2020). Systematic Investigations on Continuous Fluidized Bed Crystallization for Chiral Separation. Crystals, 10, 394.
Carneiro, T., Wrzosek, K., Bettenbrock, K., Lorenz, H., & Seidel-Morgenstern, A. (2020). Immobilization of an amino acid racemase for application in crystallization‐based chiral resolutions of asparagine monohydrate. Engineering in Life Sciences, 20, 550-561.
Lorenz, H., & Seidel-Morgenstern, A. (2020). Separation Processes to Provide Pure Enantiomers and Plant Ingredients. Annual Review of Chemical and Biomolecular Engineering, 11, 469–502.
Temmel, E., Eicke, M., Cascella, F., Seidel-Morgenstern, A., & Lorenz, H. (2019). Resolution of Racemic Guaifenesin Applying a Coupled Preferential Crystallization-Selective Dissolution Process: Rational Process Development. Crystal Growth & Design, 19, 3148-3157.
Carneiro, T., Bhandari, S., Temmel, E., Lorenz, H., & Seidel-Morgenstern, A. (2019). Shortcut Model for Describing Isothermal Batch Preferential Crystallization of Conglomerates and Estimating the Productivity. Crystal Growth & Design, 19, 5189-5203.
Bhandari S., Carneiro T., Temmel, E., Qamar, S., Lorenz, H., Seidel-Morgenstern, A. (2018). Modeling Batch Preferential Crystallization for Conglomerates and Racemic Compounds. In Proceedings of BIWIC 2018; Univ Rouen Havre: Rouen, France. 121-125.
Cascella, F., Temmel, E., Seidel-Morgenstern, A., & Lorenz, H. (2018). Fundamental Studies for Continuous Preferential Crystallization of Guaifenesin. In BIWIC2018 - 25th International Workshop on Industrial crystallization. Rouen, France. 64-69.
Bhandari, S., Temmel, E., Carneiro, T., Qamar, S., Lorenz, H., & Seidel-Morgenstern, A. (2018). Modeling Batch Preferential Crystallization for Conglomerates and Racemic Compounds. In BIWIC2018 - 25th International Workshop on Industrial crystallization. Rouen, France. 121-125.
Temmel, E., Gänsch, J., Lorenz, H., & Seidel-Morgenstern, A. (2018). Measurement and Evaluation of the Crystallization Kinetics of LAsparagine Monohydrate in the Ternary L/DAsparagine/Water System. Crystal Growth & Design, 18, 7504-7517.
Mangold, M., Khlopov, D., Temmel, E., Lorenz, H., Seidel-Morgenstern, A. (2017). Modelling geometrical and fluid-dynamic aspects of a continuous fluidized bed crystallizer for separation of enantiomers. Chem. Eng. Sci. 160, 281-290.
Kerst, K., Roloff, C., Medeiros de Souza, L.G., Bartz, A., Seidel-Morgenstern, A., Thévenin, D., Janiga, G. (2017). CFD-DEM simulations of a fluidized bed crystallizer. Chem. Eng. Sci. 165, 1-13.
Binev, D., Seidel-Morgenstern, A., Lorenz, H. (2016). Continuous separation of isomers in fluidized bed crystallizers. Cryst. Growth Des. 16, 1409-1419.
Lorenz, H.; Seidel-Morgenstern, A. (2014). Processes to separate enantiomers. Angew. Chem. Int. Ed., 53, 1218−1250.
Counter-current crystallization for the separation of solid solutions
For several reasons, a single crystallization step might not be sufficient to purify a mixture of two or more components. Possible reasons can be high purity requirements (especially for pharmaceuticals), the inclusion of impurities in the crystal lattice by kinetic effects, residual mother liquor attached to crystals after solid-liquid separation or miscibility in the solid state. Especially the latter phenomena, also known as formation of solid solutions or mixed crystals, is a challenging separation problem as the impurity results of the systems thermodynamics and, usually cannot be overcome by seeding.
Classically, fractional crystallization has to be applied to resolve solid solutions which is capable to purify the mixture up to a highly pure crystalline component by repeated dissolving and recrystallization. However, since only the solid phase is used after each crystallization step, this approach produces huge amounts of liquid waste, resulting in low process yields.
Similar to rectification or counter-current extraction, this drawback can be resolved when using a cascade of separation stages (crystallizers), in which solid and liquid phases are transported counter-currently as shown in Figure 4. The transportation of the solid phase in a stage J-1 along this cascade is guaranteed by dissolving it in the mother liquor of stage J+1. Then this solution is transported to stage J, where a new crystallization run is performed. By this sequence, known as HAPILA concept, the typically challenging solid phase transport can be realized easily via its dissolved form, producing, in an ideal case, no waste but 2 pure products A and B.
![Figure 4: Scheme of the counter-current crystallization developed by the HAPILA GmbH according to.[HAPILA GmbH, Patent DE102008023833 (B4), 2013]](/3542505/original-1636466607.jpg?t=eyJ3aWR0aCI6MjQ2LCJvYmpfaWQiOjM1NDI1MDV9--a1627891f1a057dc693ef2184a4424c6702c3c2a)
[HAPILA GmbH, Patent DE102008023833 (B4), 2013]
First works were directed to fundamental studies of the solid-liquid equilibrium of interesting inorganic and organic solid solution forming systems in water, and provided simple empirical expressions to describe the phase diagram mathematically. Recently these fundamentals were extended to solid-liquid equilibria in water/ethanol mixtures used for antisolvent crystallization. Additionally, thermodynamic models such as NRTL and PC-SAFT were used to describe the phase behavior for ternary systems empirically as well as predictively. Figure 5 shows the crystallization of solid solution forming solutes A and B in a solvent/solvent mixture C in a (pseudo-)ternary phase diagram in a counter-current cascade exemplified for the evaporative (left) and antisolvent (right) crystallization.
![Figure 5: Left: ternary phase diagram showing the process path for an evaporative crystallization. Right: pseudo-ternary phase diagram showing the process path for an antisolvent crystallization (zoomed for better visibility). (1): mixing of feed and solid; (2) mixing of solid and liquid; (3) complete dissolution, (4) left: solvent evaporation, right: antisolvent addition; (5) crystallization, (6) antisolvent removal (right only).[Tenberg, V., Sadeghi, M., Seidel-Morgenstern, A., and Lorenz, H. (2022). Bypassing thermodynamic limitations in the Crystallization-based separation of solid solutions. Sep. Pur. Technol., 283, 120169.]](/4272859/original-1643212803.jpg?t=eyJ3aWR0aCI6MjQ2LCJvYmpfaWQiOjQyNzI4NTl9--ca6ac8b801a89fcd9151aba5b2850a4f7a98f68b)
[Tenberg, V., Sadeghi, M., Seidel-Morgenstern, A., and Lorenz, H. (2022). Bypassing thermodynamic limitations in the Crystallization-based separation of solid solutions. Sep. Pur. Technol., 283, 120169.]
A flexible equilibrium mass balance model, using additional solid-liquid equilibrium information, was developed to study and optimize this complicated sequentially operated counter-current crystallization process by simulation for evaporative as well as antisolvent crystallization. Moreover, the model is used to highlight analogies to more common counter-current processes (e.g. rectification, counter-current extraction or simulated moving bed chromatography). Based on this simulation studies a pilot plant using a modified HAPILA concept was constructed to demonstrate the counter-current crystallization principle experimentally. Recently, utilities were added to enable an antisolvent recycle within the pilot plant.
References
Tenberg, V., Sadeghi, M., Seidel-Morgenstern, A., and Lorenz, H. (2022). Bypassing thermodynamic limitations in the Crystallization-based separation of solid solutions. Sep. Pur. Technol., 283, 120169.
Sadeghi, M., Tenberg, V., Münzberg, S., Lorenz, H., Seidel-Morgenstern, A. (2021). Phase Equilibria of L-Valine/L-Leucine Solid Solutions. Journal of Molecular Liquids, 340, 117315.
Münzberg, S., Vu, T. G., & Seidel ‐ Morgenstern, A. (2018). Generalizing Countercurrent Processes: Distillation and Beyond. Chemie ‐ Ingenieur ‐ Technik, 90(11), 1769 ‐ 1781.
Isakov, A. I., Kotelnikova, E. N., Münzberg, S., Bocharov, S. N., Lorenz, H. (2016). Solid phases in the system L-valine – L-isoleucine. Cryst. Growth Des. 16 , 2653 – 2661.
Münzberg, S., Lorenz, H., Seidel-Morgenstern, A. (2016). Multistage counter-current crystallization for the separation of solid solutions. Chem. Eng. Technol. 39 , 1242 – 1250.
Temmel, E., Wloch, S., Müller, U., Grawe, D., Eilers, R., Lorenz, H., and Seidel-Morgenstern, A. (2013). Chem. Eng. Sci. 114, 662–673.